Antibody fragmentation and/or disulfide bond (DSB) reduction are common phenomena in antibody production processes, mainly caused by non-enzymatic (environmental) factors and enzymatic catalysis. Compared with traditional monoclonal antibodies (mAbs), bispecific antibodies (bsAbs) have more complex molecular structures, and the introduction of additional domains can exacerbate fragmentation and/or DSB reduction occurrence. In addition, fragmentation and/or DSB reduction can also lead to antibody aggregation and enhance heterogeneous products, thereby affecting products’ immunogenicity and pharmacodynamic properties.
This article focuses on the reduction and fragmentation consequences in bsAbs production and provides an in-depth analysis of the causes: non-enzymatic factors and enzymatic catalysis. In addition, the kinetics of reduction and re-oxidation in bsAbs production was further analyzed to explore effective strategies and alleviate related problems.
Bispecific Antibodies
BsAbs are an emerging class of biotherapeutics that bind to two different epitopes. The multivalent antigen-binding ability of bsAbs significantly upregulates the efficiency and has a wide range of applications, including in the treatment of lupus, rheumatoid arthritis, osteoporosis, lymphoma, leukemia, and other diseases.
There are various formats of bsAbs, and more than 100 bsAbs have been reported, each with a unique combination of antigen-binding domains. One of the bsAb formats contains a recombinant single-chain fragment variable (scFv) conjugated to a full-length IgG heavy chain, forming an IgG-scFv fusion protein (IgG-scFv Fusion). Additional scFvs contain antigen-binding VH and VL domains, typically linked together with glycine or serine repeat peptides, as well as engineered DSBs to improve biochemical stability.
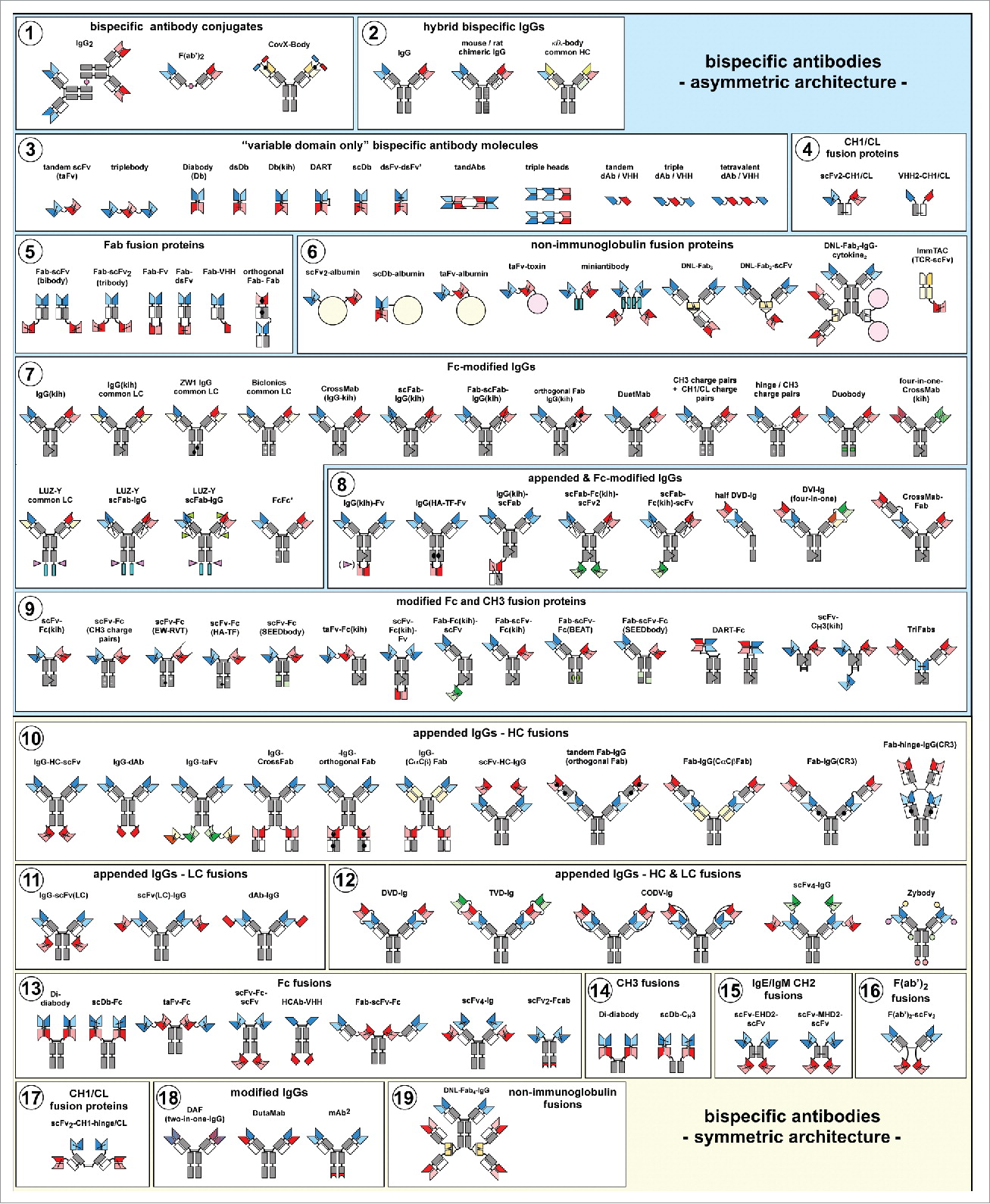
Fig. 1 The zoo of bispecific antibody formats Overview of bispecific antibody formats reduced to practice, grouped into molecules with symmetric or asymmetric architecture. (Brinkmann, 2017)
Reduction and Fragmentation of BsAbs
Although the stability of BsAb can be improved by various engineering means, the introduction of additional scFv still raised challenges during the production process. Moreover, since BsAb is a kind of mAbs in nature, the instability consequences related to mAb are also reflected in BsAb, and the mAb related challenges have been studied thoroughly, among which the main unstable forms are aggregates and fragments. In addition to stability challenges similar to mAb production, BsAb-unique stability challenges are also introduced due to the higher complexity of BsAbs. For example, in the IgG-scFv fusion molecule, additional domains are added by scFv, and the relative position of scFv in the BsAb molecule has an important impact on the overall stability of the molecule. Moreover, the biological activity of BsAbs is reduced due to the possibility of intermolecular DSBs formation by engineered DSBs between the VH and VL domains of scFv, which in turn form monomers or dimers. Nevertheless, the relative position of scFv in BsAb or intermolecular DSB can also lead to molecular conformation and protein colloid changes, which ultimately affect the pharmacokinetic and pharmacodynamic properties of the antibody.
- Non-enzymatic factors
Process conditions such as protein concentration, hydrophobicity, ionic strength, surface charge, solution pH, excipients, agitation, freeze-thaw and temperature all contribute to the stability of BsAb. Although protein backbones are very stable under physiological conditions, the presence of certain amino acid sequences (reactive side chains), solvent conditions (e.g., pH and temperature), and impurities (e.g., metals and free radicals) may cause protein reduction or cleavage. Thus, the flexibility of the protein backbone and reactive side-chain residues may adversely affect protein stability. Generally, it is considered that the hinge region connecting Fab and Fc on mAbs and BsAbs is one of the most flexible regions of the molecule, which consists of the upper (EPKSCDKTHT), middle (CPPCP), and lower (APELLGP). The two most common cleavage mechanisms with proteins are peptide bond hydrolysis and β-elimination, with serine and Asp-Lys bonds of the upper hinge region being more susceptible to cleavage by β-elimination and peptide bond hydrolysis. Likewise, if the lower hinge region has a larger flexibility region or its solvent-exposed region contains Gly-Gly bonds, it is also prone to breakage under acidic and basic conditions. When Asp, Gly, Ser, Thr, Cys, or Asn residues are present in the primary sequence, the likelihood of antibody cleavage is upregulated because the side chains of all these residues (except Gly) can facilitate cleavage by specific mechanisms. In addition to the breakage of the hinge region, the (Gly)4Ser junction region of IgG-scFv Fusion is also prone to breakage.
- Enzymatic catalysis
Typically, shear forces during upstream harvesting operations cause cell lysis, releasing intracellular reductase that allows BsAb reduction.
The glutathione system includes glutathione, glutathione reductase (GR), and glutaredoxin (Grx), which are present in all mammalian cells. The glutathione system is involved in various cellular functions, including antioxidant detoxification, regulation of nitric oxide cycle, DNA synthesis, repairing metabolic-related and biochemical reactions, protein synthesis, amino acid transport, iron metabolism, cell cycle regulation, etc. On the other hand, the thioredoxin system includes thioredoxin 1 (Trx1), thioredoxin reductase 1 (TrxR1), and reduced coenzyme II (NADPH), which are used in many reduction reactions. Hydrogen donors, especially nucleoside diphosphates, are also present as domains in disulfide isomerases in converting nucleoside diphosphates to the corresponding deoxygenated products and in light-dependent reduction reactions. In addition, Trx1 generally promotes the reduction of other proteins through cysteine thiol-disulfide exchange, thereby acting as an antioxidant.
The glutathione system and the thioredoxin system play important roles in the regulation of antibody reduction. Moreover, these two systems are actively presented in cells and significantly impact cellular functions. However, upon antibody reduction, related fragments may be reconstituted into intact antibodies under oxidative conditions with no significant changes in biological activities. According to studies, during the virus inactivation step with low pH conditions for antibody purification, reduced antibodies in the harvested cell culture medium may aggregate and cause unintended immune responses in patients or affect the therapeutic properties of drugs. Therefore, it is necessary to investigate the sensitivity of BsAbs to cellular reductases and the resulting impurities to improve product quality and process robustness accordingly.
BsAb Reduction/Re-oxidation Kinetics
Studying the extracellular or in vitro reduction and re-oxidation of BsAb can provide insight into the redox kinetics and explore strategies for BsAb reduction and fragmentation-related challenges. In 2020, Swope et al. investigated the in vitro reduction/re-oxidation kinetics of BsAb (IgG-scFv Fusion) using the thioredoxin system. In addition, since mAb reduction is associated with aggregation under low pH virus inactivation step for antibody purification, this study also evaluated the BsAb enzymatic activity under different conditions, including low pH treatment, without low pH treatment, and at room temperature to evaluate re-oxidation activities after reduction.
- Reduction kinetics and fragmentation pathways of BsAbs
The reduction behavior of BsAbs in vitro was investigated using the recombinant TrxR1 enzyme. Results demonstrated that the percentage of each intact molecule (LHHL) could be fitted to a first-order exponential decay function to determine the sensitivity of BsAbs and parental mAbs to TrxR1. These results suggest that reduction occurs rapidly, and there is no steric competition for reducing thioredoxin by additional interdomain DSBs on the single-chain antibody. Furthermore, various intermediates are formed during the reduction and re-oxidation process of antibodies, including light chain-deficient antibodies (LHH), heavy chain dimers (HH), and half-antibody species (LH). In addition, NR-CE could not detect the reduction of DSBs of single-chain antibodies between BsAb domains because they were located within the BsAb heavy chain; therefore, upon reduction of DSBs of single-chain antibody by NR-CE, the overall molecular weight of BsAb’s heavy chain remained similar.
- Re-oxidation process after reduction of BsAb thioredoxin
In addition to characterizing the reduction behavior of BsAb, the impurity distribution upon re-oxidation was also investigated. Although in vitro re-oxidation studies cannot fully replicate all cell culture conditions, harvest, and storage, impurity formation due to BsAb reduction can be studied using controlled environmental conditions. Experimentally, BsAb and parental mAb were reduced under the thioredoxin system and then re-oxidized in vials containing air at room temperature for 30 days. As a result, it demonstrated that most BsAbs have higher aggregation potential after re-oxidation than that of the parental mAbs.
To further characterize the re-oxidation pattern of antibodies, antibody species from the end of oxidation were assessed. Ideally, the re-oxidation of interchain DSBs of mAb will generate fully oxidized molecule LHHL and partially re-oxidized molecules including LHH, HH, and LH. The latter three partially re-oxidized molecules are typical fragments that meet expectations and can be identified using NR-CE. Moreover, typical fragments other than these 4 species are uniformly classified as “new heteroplasms.” In contrast with re-oxidized and partially reduced samples, the reduced mAb formed a new heterogeneous form, the light chain dimer (LL), after 30 days of storage. In addition to LL, there are other new isoforms of lower content. Further CE analysis (R-CE) under reducing conditions revealed that the presence of the novel heterogeneous body was caused by DSBs mismatch. Moreover, LL can be removed using conventional protein A capture but may downregulate overall product yields. In contrast, BsAbs generated 5 novel isoforms unrelated to the theoretical molecular weights of typical antibody fragments (LHH, HH, LH) and/or intact BsAbs (LHHL), including a heterogeneity with molecular weight between LH and HH. Similarly, R-CE analysis revealed that the new heteroform after BsAb re-oxidation was also caused by DSB mismatches. The additional single-chain antibody fragment resulted in more BsAb mismatches than the parental mAb. Mass spectrometry analysis demonstrates that there were no other cleavage fragments, e.g., single-chain antibody cleavage from BsAb, were formed in the re-oxidized sample.
- Re-Oxidation of BsAb at Low pH
Low pH viral inactivation is a common step in antibody purification and may affect antibody re-oxidation and aggregation behavior. In this study, antibodies were reduced using the thioredoxin system and treated under low pH, and then antibodies were neutralized subsequently to determine the effect of low pH viral inactivation on aggregate formation with reduced BsAb samples. Specifically, after complete reduction with thioredoxin, reaction pH was adjusted to 3.5 with acetic acid and neutralized to pH 7.4 with Tris base. Then, the reaction was re-oxidized at room temperature for 30 days.
Low pH treated samples did not significantly increase the level of aggregation after re-oxidation compared to the aforementioned samples without using low pH treatment. Furthermore, new allosome portions that emerged after re-oxidation of the low pH/neutralized antibody were also reduced. For example, LL was not observed in samples with lower pH values. The reason for this is speculated to be that the electrostatic surface properties of antibodies at low pH lead to different aggregation and re-oxidation behaviors. At pH 3.5, light and heavy chain fragments of BsAb and the parent mAb presented a large number of positively charged surface residues from protonated carboxylic acid side chains. Therefore, under low pH conditions, electrostatic repulsion between antibody fragments prevents the formation of new heterosomes and aggregates.
In conclusion, BsAbs and parental mAbs showed similar sensitivity to thioredoxin reduction, and antibody formats affect intermediates formations during the enzymatic reduction process. In addition, BsAbs produced a more variety of impurities and higher contention proportions during the re-oxidation process. Moreover, the formation of impurities after re-oxidation is related to the sample processing conditions. Based on these results, it is suspected that the storage of harvest solutions containing bsAb reduction products without immediate purification after harvest may lead to protein aggregates and DSB mismatches. Moreover, immediate purification does not completely prevent the formation of impurity fragments due to disulfide mismatches under oxidative conditions. There are many kinds of impurities formed during the re-oxidation of the sample, and impurity removed remained a challenging task; thus, the use of engineered DSB should be avoided.
Perspectives
BsAbs are structurally complex, and the introduction of new domains into mAbs, especially DSB-containing domains, may further advance the molecule’s complexity, thereby exacerbating its reduction and/or fragmentation challenges. While the molecular nature is the determining factor of this question, environmental factors can also influence reduction and/or fragmentation to some extent. According to the information provided by this review, you can try to optimize from the following aspects to alleviate the BsAb reduction and/or fragmentation-related problems.
The flexibility of the protein backbone and reactive side chains can lead to adverse effects on protein stability, and the presence of these regions allows molecules to influence protein concentration, hydrophobicity, ionic strength, surface charge, solution pH, excipients, stirring, freezing and thawing temperature, and other process conditions to become more sensitive. Therefore, on the one hand, the introduction of such a region can be avoided during molecular screening; on the other hand, the process mentioned above conditions can be reasonably set and optimized according to the sensitivity characteristics of the molecule so as to improve the stability of the molecule.
DSBs are important contributors to reduction and/or fragmentation challenges. In molecular design, DSBs can be minimized or lessened accessibility to avoid exposure of DSBs to reducing environmental conditions. In the process of cell culture, it is necessary to balance cell process performance and product quality, prevent the use of reducing substances while maintaining process performance, and ensure sufficient oxygen supply. In addition, other process optimization can also be adopted to improve cell viability avoid cell death and rupture during cell culture, thereby reducing the release of intracellular substances or enzymes. When harvesting, use a harvesting method with low shear force and pass in air to avoid creating a reducing environment or causing cell death. In addition, it is necessary to purify in time to prevent reduction and re-oxidation during downstream process optimization, resulting in more impurities. Moreover, purification strategies, step sequences, and process parameters can be rationally designed according to molecular characteristics, including removing antibody reduction products or fragments in advance, adding redox pairs to the washing solution to protect antibody disulfide bonds, etc.
In addition, with the continuous development of computer-aided molecular design technology, predicting the stability of molecules based on molecular sequences become possible in the future, thus preventing the introduction of instability factors from the molecular screening stage.
Essentially, BsAb is a kind of mAb. Although related research on the reduction and/or fragmentation of BsAb is still limited, research on mAb is relatively thorough. Therefore, in addition to solving the unique problems of BsAb from the above aspects, the historical studies of mAb can also be referred to avoid certain instability challenges and improve the production efficiency of bsAbs.
Reference
1. Brinkmann, Ulrich, and Roland E. Kontermann. “The making of bispecific antibodies.” MAbs. Vol. 9. No. 2. Taylor & Francis, 2017.